
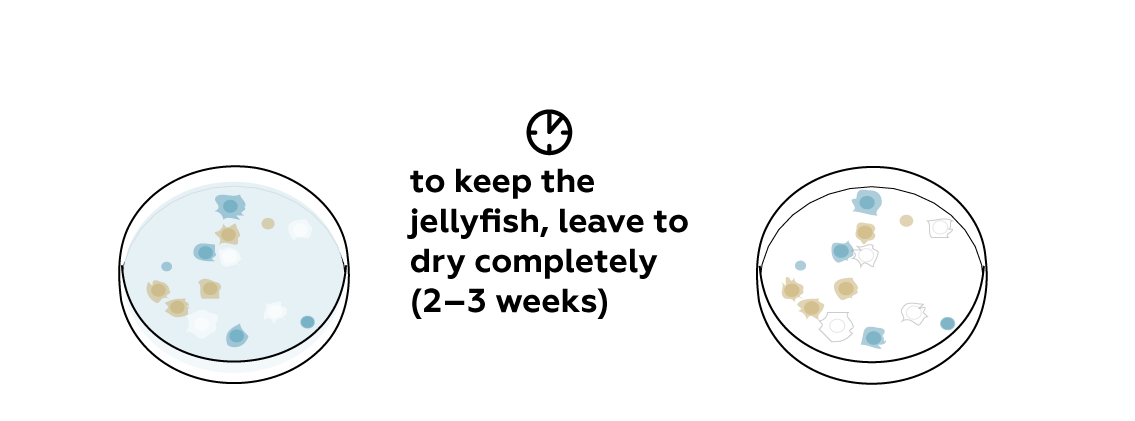
Various strategies have been developed to tackle the above issues, including designing hierarchical electrode structures 19, 20, 21, 22, 23, 24, 25, modifying electrolyte formulations 26, 27, 28, 29, 30, and optimizing charge/discharge protocols 31, 32. This would finally give rise to a low reversibility for the Zn deposition/stripping process. Higher cycling capacity would bring about greater volume change, which leads to the pulverization of Zn foil and may make Zn dendrites lose contact with the electrodes, becoming “dead Zn” 13, 18. Subsequently, the dendrites are formed and quickly render a short circuit of cells. Specifically, at higher current densities, the Zn 2+ around the electrode/electrolyte interface are rapidly depleted 15, 16, 17. In particular, high current density and large cycling capacity significantly exacerbate the formation of Zn dendrites, thus dramatically restricting the power and energy density of Zn metal batteries 14. The formed Zn protrusions further increase the local electric field intensity around them, leading to the evolution of Zn protuberances into Zn dendrites upon cycling. Therefore, zinc nucleation and growth prefer to occur at such tips (i.e., “tip effect”) 13, resulting in inhomogeneous Zn deposition. In addition, both Zn 2+ and the electric field tend to concentrate at the protuberances with high surface energy 13.
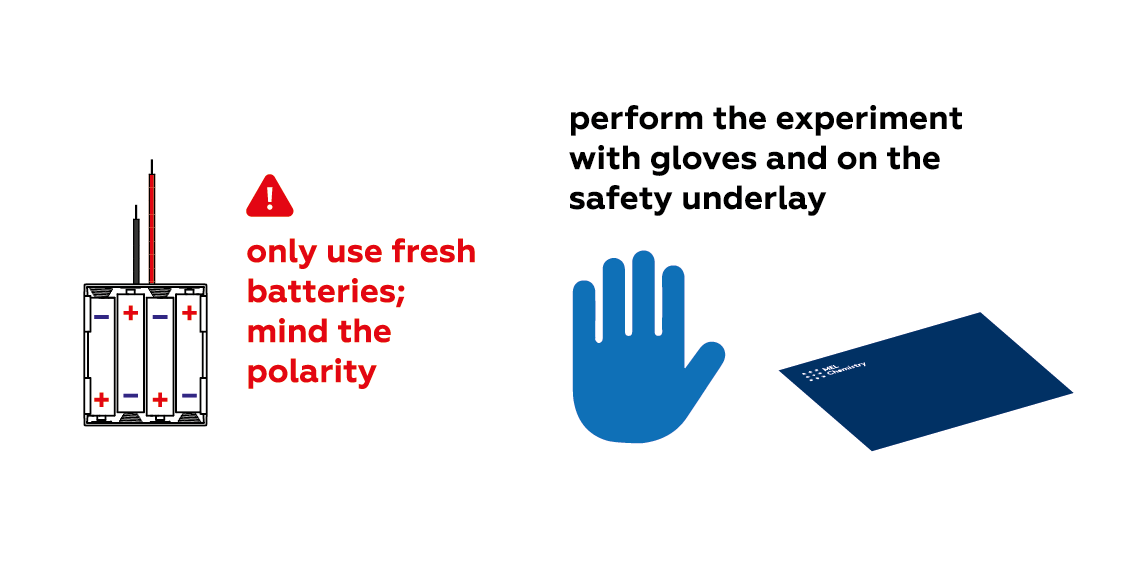

It is well known that during Zn deposition, an uneven electric field distribution inevitably forms at the pores of the separator (Fig. Although primary Zn metal batteries (e.g., Zn-MnO 2 cells) have been commercialized and used, the practical application in rechargeable batteries is hindered by the formation of Zn dendrites 9, 10. Among various aqueous batteries, zinc metal batteries have attracted extensive attention, because Zn anodes are prized for high volumetric capacity (5851 mAh/cm 3), abundant resources, and environmental friendliness 6, 7, 8. Besides, high ionic conductivities of aqueous media result in superior power densities 5. The utilization of water solvent offers the advantages of low cost, high safety, and environmental benignity 3, 4. Furthermore, the concept could be readily extended to sodium metal anodes, demonstrating the interfacial chemistry regulation of separator is a promising route to circumvent the metal anode challenges.Īqueous rechargeable batteries are promising alternatives to conventional Li-ion batteries for large-scale energy storage systems 1, 2. Consequently, dendrite-free zinc morphologies and superior cycling stability are achieved at simultaneous high current densities and large cycling capacities (1000 h at 5 mA/cm 2 for 5 mAh/cm 2 and 500 h at 10 mA/cm 2 for 10 mAh/cm 2).
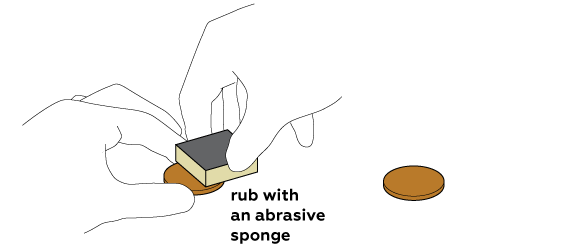
The former homogenizes the electric field distribution for smooth zinc metal on the substrate, while the latter enables the concurrent zinc deposition on the separator with a face-to-face growth. Here we turn to regulate the separator’s interfacial chemistry through tin coating with decent conductivity and excellent zincophilicity. Tailoring the deposition behavior on the substrate could partly resolve dendrites’ formation, but it usually works only under low current densities and limited capacities. Stable plating/stripping of metal electrodes under high power and high capacity remains a great challenge.
